High-Temperature Materials for Thermionic Energy Conversion
Thermionic energy converters are based on the emission of electrons from a hot cathode and their collection by a cooler anode. In this process, they convert heat directly into electricity and have the potential to achieve high efficiencies comparable to those of conventional heat engines. As a part of a highly collaborative project (with Prof. Howe at Stanford, Prof. Igor Bargatin at Penn, and Spark Thermionics), we are developing a microfabricated, close-gap thermionic energy converter for directly converting heat from a combustion source into electricity. One key challenge is associated with the cathode, which needs to be highly conductive and survive temperatures as hot as 2000 °C in an oxidizing environment. We are developing processes for fabricating a SiC-protected W cathode and investigating its long-term stability under harsh environments.Material Science of Concrete towards Green Construction
Production of concrete based on Portland cement (PC) hydration is an energy and water intensive process responsible for a staggering 5-8% of the annual global CO2 emissions. Most concrete is manufactured using PC clinker, which is mainly produced by reacting limestone (lime bearing), clay (silica, alumina, and iron), and other ingredients such as alumina and iron oxide at temperatures up to 1450 °C. The main reaction product of PC hydration is calcium silicate hydrate (C-S-H) gel, which precipitates as nanometer sized particles containing a polymerized layered structure. C-S-H acts as the primary binding phase in the hydrated PC matrix. In modern PC-based concrete, aluminum is incorporated into the C-S-H structure (C-A-S-H), by substituting PC with Al-bearing supplementary cementitious materials (SCMs) such as fly ash. The valorization of industrial by-product SCMs in cement manufacturing is an effective practice towards sustainable development. Our research, in collaboration with Prof. Monteiro’s group, aims to understand the chemistry and structure of C-(A-)S-H in order to obtain concrete of desired quality and to reduce the carbon footprint of the cement industry.
Recently Completed Projects
High Surface Area Nanomaterials for Supercapacitors
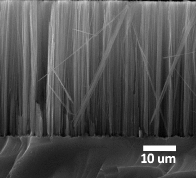